The Open Field Test
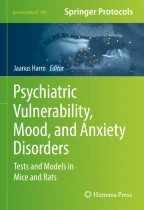
The open field test (OFT) is the most widely used method for observing the behavior of mice and rats under laboratory conditions. It is used to evaluate the exploratory behavior, general locomotor activity and emotionality of rats and mice. However, it has to be kept in mind that these behaviors are not independent; they interact and so a change in one will affect another. Despite being a seemingly simple test, several caveats need to be acknowledged when selecting the variables for analysis and interpreting the data with regard to the aforementioned domains. The observed behaviors can depend on a number of procedural, environmental, and biological factors, which should be carefully considered when planning the experiment, as well as during the analysis and interpretation of the results. This review provides a critical overview of these factors, followed by some warnings and practical tips for conducting the OFT.
This is a preview of subscription content, log in via an institution to check access.
Access this chapter
Subscribe and save
Springer+ Basic
€32.70 /Month
- Get 10 units per month
- Download Article/Chapter or eBook
- 1 Unit = 1 Article or 1 Chapter
- Cancel anytime
Buy Now
Price includes VAT (France)
eBook EUR 149.99 Price includes VAT (France)
Softcover Book EUR 189.89 Price includes VAT (France)
Hardcover Book EUR 210.99 Price includes VAT (France)
Tax calculation will be finalised at checkout
Purchases are for personal use only
Similar content being viewed by others
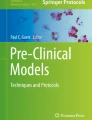
The Open Field Test for Measuring Locomotor Activity and Anxiety-Like Behavior
Chapter © 2019
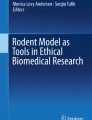
Assessment of Motor Function in Rodents: Behavioral Models Sharing Simplicity and Multifaceted Applicability
Chapter © 2016
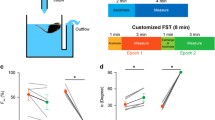
Novel insights into the behavioral analysis of mice subjected to the forced-swim test
Article Open access 14 April 2015
References
- Hall CS (1934) Emotional behaviour in the rat: I. Defecation and urination as measures of individual differences in emotionality. J Comp Psychol 18:385–403 ArticleGoogle Scholar
- Hall CS (1936) Emotional behavior in the rat. III. The relationship between emotionality and ambulatory activity. J Comp Psychol 22(3):345–352 ArticleGoogle Scholar
- Hall CS (1937) Emotional behavior in the rat. IV. The relationship between emotionality and stereotyping of behavior. J Comp Psychol 24(2):369–375 ArticleGoogle Scholar
- Broadhurst PL (1957) Determinants of emotionality in the rat: I. Situational factors. Br J Psychol 48(1):1–12 ArticleCASPubMedGoogle Scholar
- Broadhurst PL (1958) Determinants of emotionality in the rat: II. Antecedent factors. Br J Psychol 49(1):12–20 ArticleCASPubMedGoogle Scholar
- Broadhurst PL (1958) Determinants of emotionality in the rat: III. Strain differences. J Comp Physiol Psychol 51(1):55–59 ArticleCASPubMedGoogle Scholar
- Broadhurst PL (1975) The Maudsley reactive and nonreactive strains of rats: a survey. Behav Genet 5(4):299–319 ArticleCASPubMedGoogle Scholar
- Blizard DA, Adams N (2002) The Maudsley reactive and nonreactive strains: a new perspective. Behav Genet 32(5):277–299 ArticlePubMedGoogle Scholar
- McQuade R, Stanford SC (2001) Differences in central noradrenergic and behavioural responses of Maudsley non-reactive and Maudsley reactive inbred rats on exposure to an aversive novel environment. J Neurochem 76(1):21–28 ArticleCASPubMedGoogle Scholar
- Royce JR (1977) On the construct validity of open-field measures. Psychol Bull 84(6):1098–1106 ArticleGoogle Scholar
- Roth KA, Katz RJ (1979) Stress, behavioral arousal, and open field activity–a reexamination of emotionality in the rat. Neurosci Biobehav Rev 3(4):247–263 ArticleCASPubMedGoogle Scholar
- Archer J (1973) Tests for emotionality in rats and mice: a review. Anim Behav 21(2):205–235 ArticleCASPubMedGoogle Scholar
- Denenberg VH (1969) Open-field behavior in the rat: what does it mean? Ann N Y Acad Sci 159(3):852–859 ArticleCASPubMedGoogle Scholar
- Prut L, Belzung C (2003) The open field as a paradigm to measure the effects of drugs on anxiety-like behaviors: a review. Eur J Pharmacol 463(1–3):3–33 ArticleCASPubMedGoogle Scholar
- Brown RE, Stanford L, Schellinck HM (2000) Developing standardized behavioral tests for knockout and mutant mice. ILAR J 41(3):163–174 ArticleCASPubMedGoogle Scholar
- Crawley JN (1999) Behavioral phenotyping of transgenic and knockout mice: experimental design and evaluation of general health, sensory functions, motor abilities, and specific behavioral tests. Brain Res 835(1):18–26 ArticleCASPubMedGoogle Scholar
- Hanell A, Marklund N (2014) Structured evaluation of rodent behavioral tests used in drug discovery research. Front Behav Neurosci 8:252 PubMedPubMed CentralGoogle Scholar
- Crawley JN (2000) What’s wrong with my mouse? Behavioral phenotyping of transgenic and knockout mice. Wiley-Liss, New York Google Scholar
- Stanford SC (2007) The open field test: reinventing the wheel. J Psychopharmacol 21(2):134–135 ArticlePubMedGoogle Scholar
- Stanford SC (2007) Open fields (unlike wheels) can be any shape but still miss the target. J Psychopharmacol 21(2):144 ArticlePubMedGoogle Scholar
- Blizard DA et al (2007) Test standardization in behavioural neuroscience: a response to Stanford. J Psychopharmacol 21(2):136–139 ArticlePubMedGoogle Scholar
- Rodgers RJ (2007) More haste, considerably less speed. J Psychopharmacol 21(2):141–143 ArticleCASPubMedGoogle Scholar
- Fonio E, Golani I, Benjamini Y (2012) Measuring behavior of animal models: faults and remedies. Nat Methods 9(12):1167–1170 ArticleCASPubMedGoogle Scholar
- Whishaw IQ et al (2001) Accelerated nervous system development contributes to behavioral efficiency in the laboratory mouse: a behavioral review and theoretical proposal. Dev Psychobiol 39(3):151–170 ArticleCASPubMedGoogle Scholar
- Whishaw IQ, Tomie JA (1996) Of mice and mazes: similarities between mice and rats on dry land but not water mazes. Physiol Behav 60(5):1191–1197 ArticleCASPubMedGoogle Scholar
- Whishaw IQ (1995) A comparison of rats and mice in a swimming pool place task and matching to place task: some surprising differences. Physiol Behav 58(4):687–693 ArticleCASPubMedGoogle Scholar
- O’Leary TP, Gunn RK, Brown RE (2013) What are we measuring when we test strain differences in anxiety in mice? Behav Genet 43(1):34–50 ArticlePubMedGoogle Scholar
- Takahashi A et al (2006) Multivariate analysis of temporal descriptions of open-field behavior in wild-derived mouse strains. Behav Genet 36(5):763–774 ArticlePubMedGoogle Scholar
- Drai D et al (2001) Rats and mice share common ethologically relevant parameters of exploratory behavior. Behav Brain Res 125(1–2):133–140 ArticleCASPubMedGoogle Scholar
- Suarez SD, Gallup GG (1981) An ethological analysis of open-field behavior in rats and mice. Learn Motiv 12(3):342–363 ArticleGoogle Scholar
- Welker WI (1957) “Free” versus “forced” exploration of a novel situation by rats. Psychol Rep 3(1):95–108 ArticleGoogle Scholar
- Fonio E, Benjamini Y, Golani I (2012) Short and long term measures of anxiety exhibit opposite results. PLoS One 7(10):e48414 ArticleCASPubMedPubMed CentralGoogle Scholar
- Bolivar VJ et al (2000) Habituation of activity in an open field: a survey of inbred strains and F1 hybrids. Behav Genet 30(4):285–293 ArticleCASPubMedGoogle Scholar
- Leussis MP, Bolivar VJ (2006) Habituation in rodents: a review of behavior, neurobiology, and genetics. Neurosci Biobehav Rev 30(7):1045–1064 ArticlePubMedGoogle Scholar
- Bolivar VJ (2009) Intrasession and intersession habituation in mice: from inbred strain variability to linkage analysis. Neurobiol Learn Mem 92(2):206–214 ArticlePubMedPubMed CentralGoogle Scholar
- Lipkind D et al (2004) New replicable anxiety-related measures of wall vs center behavior of mice in the open field. J Appl Physiol 97(1):347–359 ArticlePubMedGoogle Scholar
- Angrini M, Leslie JC, Shephard RA (1998) Effects of propranolol, buspirone, pCPA, reserpine, and chlordiazepoxide on open-field behavior. Pharmacol Biochem Behav 59(2):387–397 ArticleCASPubMedGoogle Scholar
- Salmon P, Stanford SC (1989) Beta-adrenoceptor binding correlates with behaviour of rats in the open field. Psychopharmacology 98(3):412–416 ArticleCASPubMedGoogle Scholar
- Lindquist MP, Götestam KG (1977) Open-field behavior after intravenous amphetamine analogues in rats. Psychopharmacology 55(2):129–133 ArticleCASPubMedGoogle Scholar
- Zimcikova E et al (2017) Behavioral effects of antiepileptic drugs in rats: are the effects on mood and behavior detectable in open-field test? Seizure 52:35–40 ArticlePubMedGoogle Scholar
- Thompson SM, Berkowitz LE, Clark BJ (2018) Behavioral and neural subsystems of rodent exploration. Learn Motiv 61:3–15 ArticlePubMedGoogle Scholar
- Eilam D, Golani I (1989) Home base behavior of rats (Rattus norvegicus) exploring a novel environment. Behav Brain Res 34(3):199–211 ArticleCASPubMedGoogle Scholar
- van Abeelen JHF (1970) Genetics of rearing behavior in mice. Behav Genet 1(1):71–76 ArticlePubMedGoogle Scholar
- van Abeelen JHF, van der Kroon PHW, Bekkers MFJ (1973) Mice selected for rearing behavior: some physiological variables. Behav Genet 3(1):85–90 ArticlePubMedGoogle Scholar
- Lever C, Burton S, O’Keefe J (2006) Rearing on hind legs, environmental novelty, and the hippocampal formation. Rev Neurosci 17(1–2):111–133 PubMedGoogle Scholar
- Sturman O, Germain PL, Bohacek J (2018) Exploratory rearing: a context- and stress-sensitive behavior recorded in the open-field test. Stress:21(5):443–452. https://doi.org/10.1080/10253890.2018.1438405
- Delprato A et al (2017) QTL and systems genetics analysis of mouse grooming and behavioral responses to novelty in an open field. Genes Brain Behav 16(8):790–799 CASPubMedPubMed CentralGoogle Scholar
- Estanislau C (2012) Cues to the usefulness of grooming behavior in the evaluation of anxiety in the elevated plus-maze. Psychol Neurosci 5:105–112 ArticleGoogle Scholar
- Fernández-Teruel A, Estanislau C (2016) Meanings of self-grooming depend on an inverted U-shaped function with aversiveness. Nat Rev Neurosci 17(9):591–591 ArticlePubMedGoogle Scholar
- Kalueff AV et al (2016) Neurobiology of rodent self-grooming and its value for translational neuroscience. Nat Rev Neurosci 17(1):45–59 ArticleCASPubMedGoogle Scholar
- van den Boom BJG et al (2017) Automated classification of self-grooming in mice using open-source software. J Neurosci Methods 289:48–56 ArticlePubMedGoogle Scholar
- Spruijt BM, van Hooff JA, Gispen WH (1992) Ethology and neurobiology of grooming behavior. Physiol Rev 72(3):825–852 ArticleCASPubMedGoogle Scholar
- Lester D (1968) The effect of fear and anxiety on exploration and curiosity: toward a theory of exploration. J Gen Psychol 79(1):105–120 ArticleCASPubMedGoogle Scholar
- Barnett S, Cowan P (1976) Activity, exploration, curiosity and fear: an ethological study. Interdiscip Sci Rev 1:43–62 ArticleGoogle Scholar
- van Abeelen JHF (1964) Mouse mutants studied by means of ethological methods. I. Ethogram. Genetica 34(1):79–94 ArticleGoogle Scholar
- Grant EC, Mackintosh JH (1963) A comparison of the social postures of some common laboratory rodents. Behaviour 21(3/4):246–259 Google Scholar
- Irwin S (1968) Comprehensive observational assessment: Ia. A systematic, quantitative procedure for assessing the behavioral and physiologic state of the mouse. Psychopharmacologia 13(3):222–257 ArticleCASPubMedGoogle Scholar
- Wahlsten D (2011) Mouse behavioral testing. How to use mice in behavioral neuroscience. Academic Google Scholar
- Pereira TD, Shaevitz JW, Murthy M (2020) Quantifying behavior to understand the brain. Nat Neurosci 23(12):1537–1549 ArticleCASPubMedPubMed CentralGoogle Scholar
- Mathis MW, Mathis A (2020) Deep learning tools for the measurement of animal behavior in neuroscience. Curr Opin Neurobiol 60:1–11 ArticleCASPubMedGoogle Scholar
- Wiltschko AB et al (2020) Revealing the structure of pharmacobehavioral space through motion sequencing. Nat Neurosci 23(11):1433–1443 ArticleCASPubMedPubMed CentralGoogle Scholar
- von Ziegler L, Sturman O, Bohacek J (2021) Big behavior: challenges and opportunities in a new era of deep behavior profiling. Neuropsychopharmacology 46(1):33–44 ArticleGoogle Scholar
- Sturman O et al (2020) Deep learning-based behavioral analysis reaches human accuracy and is capable of outperforming commercial solutions. Neuropsychopharmacology 45(11):1942–1952 ArticlePubMedPubMed CentralGoogle Scholar
- Hobson L et al (2020) Phenotyping in mice using continuous home cage monitoring and ultrasonic vocalization recordings. Curr Prot Mouse Biol 10(3):e80 Google Scholar
- Zucker I, Beery AK (2010) Males still dominate animal studies. Nature 465(7299):690 ArticleCASPubMedGoogle Scholar
- Beery AK, Zucker I (2011) Sex bias in neuroscience and biomedical research. Neurosci Biobehav Rev 35(3):565–572 ArticlePubMedGoogle Scholar
- Gray JA (1971) Sex differences in emotional behaviour in mammals including man: endocrine bases. Acta Psychol 35(1):29–46 ArticleCASGoogle Scholar
- Johnston AL, File SE (1991) Sex differences in animal tests of anxiety. Physiol Behav 49(2):245–250 ArticleCASPubMedGoogle Scholar
- Gray JA (1979) Emotionality in male and female rodents: a reply to Archer. Br J Psychol 70(3):425–440 ArticleCASPubMedGoogle Scholar
- Palanza P (2001) Animal models of anxiety and depression: how are females different? Neurosci Biobehav Rev 25(3):219–233 ArticleCASPubMedGoogle Scholar
- Võikar V et al (2001) Strain and gender differences in the behavior of mouse lines commonly used in transgenic studies. Physiol Behav 72(1–2):271–281 ArticleCASPubMedGoogle Scholar
- Mogil JS et al (2000) Sex differences in thermal nociception and morphine antinociception in rodents depend on genotype. Neurosci Biobehav Rev 24(3):375–389 ArticleCASPubMedGoogle Scholar
- Prendergast BJ, Onishi KG, Zucker I (2014) Female mice liberated for inclusion in neuroscience and biomedical research. Neurosci Biobehav Rev 40:1–5 ArticlePubMedGoogle Scholar
- Karp NA, Reavey N (2019) Sex bias in preclinical research and an exploration of how to change the status quo. Br J Pharmacol 176(21):4107–4118 ArticleCASPubMedGoogle Scholar
- Fritz AK, Amrein I, Wolfer DP (2017) Similar reliability and equivalent performance of female and male mice in the open field and water-maze place navigation task. Am J Med Genet C Semin Med Genet 175(3):380–391 ArticlePubMedPubMed CentralGoogle Scholar
- Clayton JA (2018) Applying the new SABV (sex as a biological variable) policy to research and clinical care. Physiol Behav 187:2–5 ArticleCASPubMedGoogle Scholar
- Clayton JA, Collins FS (2014) Policy: NIH to balance sex in cell and animal studies. Nature 509(7500):282–283 ArticlePubMedPubMed CentralGoogle Scholar
- Mogil JS (2016) Perspective: equality need not be painful. Nature 535(7611):S7–S7 ArticlePubMedGoogle Scholar
- Wittchen HU et al (2011) The size and burden of mental disorders and other disorders of the brain in Europe 2010. Eur Neuropsychopharmacol 21(9):655–679 ArticleCASPubMedGoogle Scholar
- Miller LR et al (2017) Considering sex as a biological variable in preclinical research. FASEB J 31(1):29–34 ArticleCASPubMedGoogle Scholar
- Docherty JR et al (2019) Sex: a change in our guidelines to authors to ensure that this is no longer an ignored experimental variable. Br J Pharmacol 176(21):4081–4086 ArticleCASPubMedPubMed CentralGoogle Scholar
- Tuttle AH et al (2018) Comparing phenotypic variation between inbred and outbred mice. Nat Methods 15(12):994–996 ArticleCASPubMedPubMed CentralGoogle Scholar
- Festing MF (1999) Warning: the use of heterogeneous mice may seriously damage your research. Neurobiol Aging 20(2):237–244. discussion 245-6 CASPubMedGoogle Scholar
- Festing MF (2014) Evidence should trump intuition by preferring inbred strains to outbred stocks in preclinical research. ILAR J 55(3):399–404 ArticleCASPubMedGoogle Scholar
- Logue SF et al (1997) Assessment of locomotor activity, acoustic and tactile startle, and prepulse inhibition of startle in inbred mouse strains and F1 hybrids: implications of genetic background for single gene and quantitative trait loci analyses. Neuroscience 80(4):1075–1086 ArticleCASPubMedGoogle Scholar
- Crawley JN et al (1997) Behavioral phenotypes of inbred mouse strains: implications and recommendations for molecular studies. Psychopharmacology 132(2):107–124 ArticleCASPubMedGoogle Scholar
- Holmes A et al (2003) Abnormal anxiety-related behavior in serotonin transporter null mutant mice: the influence of genetic background. Genes Brain Behav 2(6):365–380 ArticleCASPubMedGoogle Scholar
- Walsh RN, Cummins RA (1976) The open-field test: a critical review. Psychol Bull 83(3):482–504 ArticleCASPubMedGoogle Scholar
- Roubertoux PL (2015) Maintaining mice for neurobehavioral examination. NeuroMethods 100:319–333 ArticleGoogle Scholar
- Võikar V et al (2005) Long-term individual housing in C57BL/6J and DBA/2 mice: assessment of behavioral consequences. Genes Brain Behav 4(4):240–252 ArticleCASPubMedGoogle Scholar
- Kulesskaya N, Rauvala H, Võikar V (2011) Evaluation of social and physical enrichment in modulation of Behavioural phenotype in C57BL/6J female mice. PLoS One 6(9):e24755 ArticleCASPubMedPubMed CentralGoogle Scholar
- Ahlgren J, Võikar V (2019) Housing mice in the individually ventilated or open cages-does it matter for behavioral phenotype? Genes Brain Behav 18(7):e12564 ArticlePubMedPubMed CentralGoogle Scholar
- Igarashi E, Takeshita S (1995) Effects of illumination and handling upon rat open field activity. Physiol Behav 57(4):699–703 ArticleCASPubMedGoogle Scholar
- Deacon RM (2006) Housing, husbandry and handling of rodents for behavioral experiments. Nat Protoc 1(2):936–946 ArticlePubMedGoogle Scholar
- Hurst JL, West RS (2010) Taming anxiety in laboratory mice. Nat Methods 7:825–826 ArticleCASPubMedGoogle Scholar
- Andrews N, File SE (1993) Handling history of rats modifies behavioural effects of drugs in the elevated plus-maze test of anxiety. Eur J Pharmacol 235(1):109–112 ArticleCASPubMedGoogle Scholar
- File SE, Fluck E (1994) Handling alters habituation and response to stimulus change in the holeboard. Pharmacol Biochem Behav 49(3):449–453 ArticleCASPubMedGoogle Scholar
- Bohlen M et al (2014) Experimenter effects on behavioral test scores of eight inbred mouse strains under the influence of ethanol. Behav Brain Res 272:46–54 ArticleCASPubMedPubMed CentralGoogle Scholar
- Stanford C, Fillenz M, Ryan E (1984) The effect of repeated mild stress on cerebral cortical adrenoceptors and noradrenaline synthesis in the rat. Neurosci Lett 45(2):163–167 ArticleCASPubMedGoogle Scholar
- Sorge RE et al (2014) Olfactory exposure to males, including men, causes stress and related analgesia in rodents. Nat Methods 11(6):629–632 ArticleCASPubMedGoogle Scholar
- Crawley JN, Paylor R (1997) A proposed test battery and constellations of specific behavioral paradigms to investigate the behavioral phenotypes of transgenic and knockout mice. Horm Behav 31(3):197–211 ArticleCASPubMedGoogle Scholar
- McIlwain KL et al (2001) The use of behavioral test batteries: effects of training history. Physiol Behav 73(5):705–717 ArticleCASPubMedGoogle Scholar
- Blokland A et al (2012) The use of a test battery assessing affective behavior in rats: order effects. Behav Brain Res 228(1):16–21 ArticlePubMedGoogle Scholar
- Bouwknecht JA et al (2004) Effects of repeated testing in two inbred strains on flesinoxan dose-response curves in three mouse models for anxiety. Eur J Pharmacol 494(1):35–44 ArticleCASPubMedGoogle Scholar
- Paylor R et al (2006) The use of behavioral test batteries, II: effect of test interval. Physiol Behav 87(1):95–102 ArticleCASPubMedGoogle Scholar
- Võikar V, Vasar E, Rauvala H (2004) Behavioral alterations induced by repeated testing in C57BL/6J and 129S2/Sv mice: implications for phenotyping screens. Genes Brain Behav 3(1):27–38 ArticleCASPubMedGoogle Scholar
- Lapin IP (1995) Only controls: effect of handling, sham injection, and intraperitoneal injection of saline on behavior of mice in an elevated plus-maze. J Pharmacol Toxicol Methods 34(2):73–77 ArticleCASPubMedGoogle Scholar
- Hughes CW (1978) Observer influence on automated open field activity. Physiol Behav 20(4):481–485 ArticleCASPubMedGoogle Scholar
- Chesler EJ et al (2002) Identification and ranking of genetic and laboratory environment factors influencing a behavioral trait, thermal nociception, via computational analysis of a large data archive. Neurosci Biobehav Rev 26(8):907–923 ArticlePubMedGoogle Scholar
- Hossain SM, Wong BK, Simpson EM (2004) The dark phase improves genetic discrimination for some high throughput mouse behavioral phenotyping. Genes Brain Behav 3(3):167–177 ArticleCASPubMedGoogle Scholar
- Yang M, Weber MD, Crawley JN (2008) Light phase testing of social behaviors: not a problem. Front Neurosci 2(2):186–191 ArticlePubMedPubMed CentralGoogle Scholar
- Beeler JA, Prendergast B, Zhuang X (2006) Low amplitude entrainment of mice and the impact of circadian phase on behavior tests. Physiol Behav 87(5):870–880 ArticleCASPubMedGoogle Scholar
- Valentinuzzi VS et al (2000) Locomotor response to an open field during C57BL/6J active and inactive phases: differences dependent on conditions of illumination. Physiol Behav 69(3):269–275 ArticleCASPubMedGoogle Scholar
- Bodden C et al (2019) Heterogenising study samples across testing time improves reproducibility of behavioural data. Sci Rep 9(1):8247 ArticlePubMedPubMed CentralGoogle Scholar
- Festing MF (1992) The scope for improving the design of laboratory animal experiments. Lab Anim 26(4):256–268 ArticleCASPubMedGoogle Scholar
- Festing MFW (2020) The “completely randomised” and the “randomised block” are the only experimental designs suitable for widespread use in pre-clinical research. Sci Rep 10(1):17577 ArticleCASPubMedPubMed CentralGoogle Scholar
- Porter AJ et al (2015) A lack of functional NK1 receptors explains most, but not all, abnormal behaviours of NK1R-/- mice(1). Genes Brain Behav 14(2):189–199 ArticleCASPubMedPubMed CentralGoogle Scholar
- Võikar V, Gaburro S (2020) Three pillars of automated home-cage phenotyping of mice: novel findings, refinement, and reproducibility based on literature and experience. Front Behav Neurosci 14:193 ArticleGoogle Scholar
- Henriques-Alves AM, Queiroz CM (2016) Ethological evaluation of the effects of social defeat stress in mice: beyond the social interaction ratio. Front Behav Neurosci 9:364 ArticlePubMedPubMed CentralGoogle Scholar
- Morello F et al (2020) ADHD-like behaviors caused by inactivation of a transcription factor controlling the balance of inhibitory and excitatory neuron development in the mouse anterior brainstem. Transl Psychiatry 10(1):357 ArticleCASPubMedPubMed CentralGoogle Scholar
- Van der Staay FJ, Steckler T (2002) The fallacy of behavioral phenotyping without standardisation. Genes Brain Behav 1(1):9–13 ArticlePubMedGoogle Scholar
- van der Staay FJ, Arndt SS, Nordquist RE (2010) The standardization-generalization dilemma: a way out. Genes Brain Behav 9(8):849–855 ArticlePubMedGoogle Scholar
- Voelkl B et al (2020) Reproducibility of animal research in light of biological variation. Nat Rev Neurosci 21(7):384–393 ArticleCASPubMedGoogle Scholar
- Würbel H (2000) Behaviour and the standardization fallacy. Nat Genet 26(3):263 ArticlePubMedGoogle Scholar
- Kafkafi N et al (2018) Reproducibility and replicability of rodent phenotyping in preclinical studies. Neurosci Biobehav Rev 87:218–232 ArticlePubMedPubMed CentralGoogle Scholar
- Crabbe JC (2016) Reproducibility of experiments with laboratory animals: what should we do now? Alcohol Clin Exp Res 40(11):2305–2308 ArticlePubMedGoogle Scholar
- Crabbe JC, Wahlsten D, Dudek BC (1999) Genetics of mouse behavior: interactions with laboratory environment. Science 284(5420):1670–1672 ArticleCASPubMedGoogle Scholar
- Wahlsten D et al (2003) Different data from different labs: lessons from studies of gene-environment interaction. J Neurobiol 54(1):283–311 ArticlePubMedGoogle Scholar
- Wurbel H (2017) More than 3Rs: the importance of scientific validity for harm-benefit analysis of animal research. Lab Anim (NY) 46(4):164–166 ArticleGoogle Scholar
- Karp NA (2018) Reproducible preclinical research-Is embracing variability the answer? PLoS Biol 16(3):e2005413 ArticlePubMedPubMed CentralGoogle Scholar
- Bikovski L et al (2020) Lessons, insights and newly developed tools emerging from behavioral phenotyping core facilities. J Neurosci Methods 334:108597 ArticlePubMedGoogle Scholar
- Kos-Braun IC, Gerlach B, Pitzer C (2020) Science forum: a survey of research quality in core facilities. elife 9:e62212 ArticlePubMedPubMed CentralGoogle Scholar
- Võikar V (2020) Reproducibility of behavioral phenotypes in mouse models – short history with critical and practical notes. J Reprod Neurosci 1(1). https://doi.org/10.31885/jrn.1.2020.1375
- Gulinello M et al (2019) Rigor and reproducibility in rodent behavioral research. Neurobiol Learn Mem 165:106780 ArticlePubMedGoogle Scholar
- Blizard DA et al (2005) Use of a standard strain for external calibration in behavioral phenotyping. Behav Genet 35(3):323–332 ArticlePubMedGoogle Scholar
- Kilkenny C et al (2009) Survey of the quality of experimental design, statistical analysis and reporting of research using animals. PLoS One 4(11):e7824 ArticlePubMedPubMed CentralGoogle Scholar
- Prager EM et al (2011) The importance of reporting housing and husbandry in rat research. Front Behav Neurosci 5:38 ArticlePubMedPubMed CentralGoogle Scholar
- Kilkenny C et al (2010) Improving bioscience research reporting: the ARRIVE guidelines for reporting animal research. PLoS Biol 8(6):e1000412 ArticlePubMedPubMed CentralGoogle Scholar
- Leung V et al (2018) ARRIVE has not ARRIVEd: support for the ARRIVE (Animal research: reporting of in vivo experiments) guidelines does not improve the reporting quality of papers in animal welfare, analgesia or anesthesia. PLoS One 13(5):e0197882 ArticlePubMedPubMed CentralGoogle Scholar
- Percie du Sert N et al (2020) The ARRIVE guidelines 2.0: updated guidelines for reporting animal research. PLoS Biol 18(7):e3000410 ArticleCASPubMedPubMed CentralGoogle Scholar
- Percie du Sert N et al (2020) Reporting animal research: explanation and elaboration for the ARRIVE guidelines 2.0. PLoS Biol 18(7):e3000411 ArticleCASPubMedPubMed CentralGoogle Scholar
- Smith AJ et al (2018) PREPARE: guidelines for planning animal research and testing. Lab Anim 52(2):135–141 ArticleCASPubMedGoogle Scholar
- Guidelines Guiding Principles for Behavioural Laboratory Animal Science. BAP Consensus Guidelines, 2013 Google Scholar
- Voehringer P, Nicholson JR (2020) Minimum information in in vivo research. Handb Exp Pharmacol 257:197–222 ArticlePubMedGoogle Scholar
- Crusio WE et al (eds) (2013) Behavioral genetics of the mouse. Volume 1. Genetics of behavioral phenotypes, Cambridge handbooks in behavioral genetics. Cambridge University Press, Cambridge Google Scholar
- Guillén J, Steckler T (2020) Good research practice: lessons from animal care and use. Handb Exp Pharmacol 257:367–382 ArticlePubMedGoogle Scholar
- Percie du Sert N et al (2017) The experimental design assistant. PLoS Biol 15(9):e2003779 ArticlePubMedPubMed CentralGoogle Scholar
- Schellinck HM, Cyr DP, Brown RE (2010) How many ways can mouse behavioral experiments go wrong? Confounding variables in mouse models of neurodegenerative diseases and how to control them. In: Brockmann TJRHJ, Naguib M, Wynne-Edwards KE, Mitani JC, Simmons LW (eds) Advances in the study of behavior. Academic, pp 255–366 Google Scholar
- Bailoo JD, Bohlen MO, Wahlsten D (2010) The precision of video and photocell tracking systems and the elimination of tracking errors with infrared backlighting. J Neurosci Methods 188(1):45–52 ArticlePubMedPubMed CentralGoogle Scholar
- Kafkafi N et al (2003) SEE locomotor behavior test discriminates C57BL/6J and DBA/2J mouse inbred strains across laboratories and protocol conditions. Behav Neurosci 117(3):464–477 ArticlePubMedGoogle Scholar
- Wolfer DP, Lipp HP (1992) A new computer program for detailed off-line analysis of swimming navigation in the Morris water maze. J Neurosci Methods 41(1):65–74 ArticleCASPubMedGoogle Scholar
- Wolfer DP et al (2001) Extended analysis of path data from mutant mice using the public domain software Wintrack. Physiol Behav 73(5):745–753 ArticleCASPubMedGoogle Scholar
- Pennington ZT et al (2019) ezTrack: an open-source video analysis pipeline for the investigation of animal behavior. Sci Rep 9(1):19979 ArticleCASPubMedPubMed CentralGoogle Scholar
- Aguiar P, Mendonça L, Galhardo V (2007) OpenControl: a free opensource software for video tracking and automated control of behavioral mazes. J Neurosci Methods 166(1):66–72 ArticlePubMedGoogle Scholar
- Sridhar VH, Roche DG, Gingins S (2019) Tracktor: image-based automated tracking of animal movement and behaviour. Methods Ecol Evol 10(6):815–820 ArticleGoogle Scholar
- Zhang C, Li H, Han R (2020) An open-source video tracking system for mouse locomotor activity analysis. BMC Res Notes 13(1):48 ArticlePubMedPubMed CentralGoogle Scholar
- Dixon LK, DeFries JC (1968) Effects of illumination on open-field behavior in mice. J Comp Physiol Psychol 66(3, Pt.1):803–805 ArticleGoogle Scholar
- McReynolds WE, Weir MW, DeFries JC (1967) Open-field behavior in mice: effect of test illumination. Psychon Sci 9(5):277–278 ArticleGoogle Scholar
- Valle FP (1970) Effects of strain, sex, and illumination on open-field behavior of rats. Am J Psychol 83(1):103–111 ArticleCASPubMedGoogle Scholar
- Kulesskaya N, Võikar V (2014) Assessment of mouse anxiety-like behaviour in the light-dark box and open-field arena: role of equipment and procedure. Physiol Behav 133:30–38 ArticleCASPubMedGoogle Scholar
- Turner JG et al (2005) Hearing in laboratory animals: strain differences and nonauditory effects of noise. Comp Med 55(1):12–23 CASPubMedGoogle Scholar
- Kraeuter AK, Guest PC, Sarnyai Z (2019) The open field test for measuring locomotor activity and anxiety-like behavior. Methods Mol Biol 1916:99–103 ArticleCASPubMedGoogle Scholar
- Seibenhener ML, Wooten MC (2015) Use of the open field maze to measure locomotor and anxiety-like behavior in mice. J Vis Exp 96:e52434 Google Scholar
- Genzel L (2021) How to control behavioral studies for rodents—Don’t project human thoughts onto them. eNeuro 8(1):ENEURO.0456-20.2021 ArticlePubMedPubMed CentralGoogle Scholar
- Hascoet M, Bourin M, Dhonnchadha BA (2001) The mouse light-dark paradigm: a review. Prog Neuro-Psychopharmacol Biol Psychiatry 25(1):141–166 ArticleCASGoogle Scholar
- Bate ST, Clark RA, Stanford SC (2017) Using InVivoStat to perform the statistical analysis of experiments. J Psychopharmacol 31(6):644–652 ArticlePubMedGoogle Scholar
- Stanford SC (2017) Confusing preclinical (predictive) drug screens with animal ‘models’ of psychiatric disorders, or ‘disorder-like’ behaviour, is undermining confidence in behavioural neuroscience. J Psychopharmacol 31(6):641–643 ArticleCASPubMedGoogle Scholar
- Stanford SC (2020) Some reasons why preclinical studies of psychiatric disorders fail to translate: what can be rescued from the misunderstanding and misuse of animal ‘models’? Altern Lab Anim 48:106–115 ArticlePubMedGoogle Scholar
Acknowledgments
Vootele Võikar is supported by Jane and Aatos Erkko Foundation.